A recent study published in the Journal of Medical Virology reviewed the current progress on three-dimensional (3D) interactions between host and viral genomes.

Background
Deoxyribonucleic acid (DNA), the genetic material, is folded delicately into extensively condensed structures called chromosomes, which are stored in the cellular nucleus. The length of genomic DNA of a somatic cell from humans is two meters wide, while the nucleus (in most cells) is less than 10 μM in diameter. Biologists have been trying to understand the DNA folding process. Early research was primarily centered around one- and two-dimensional levels of the genome.
Nevertheless, with the completion of the Human Genome Project and the encyclopedia of DNA, researchers realized that the regulation of sub-cellular processes (like gene transcription) could not be explained with one- or two-dimensional levels of the genome. The 3D structure of the genome is involved in vital regulatory processes such as DNA replication/repair, gene transcription, and cell division and differentiation.
Different approaches have been developed to investigate the interactions between viral and host genomes. The rapid development of epigenetic techniques led to the unraveling of host-virus interactions. For instance, viral proteins could hijack host cell regulatory systems; viruses also affect cellular differentiation by influencing the remodeling process(es) of the cell’s microenvironment.
Although it is known that the viral genome significantly affects the spatial structure of the host genome upon entry, resulting in its integration into the host genome, the precise positional relationship between host and viral genomes in the 3D space is understudied. In the present review, researchers summarized the current progress of the 3D genome and associated technologies.
3D genome technologies
Microscopic investigations were used in the early 3D genome studies; however, they could not help delineate the general principles of the nuclear organization due to limited throughput and resolution. On the other hand, the chromatic conformation capture (3C) technology, which was developed to quantitate the frequency of interactions of two genomic loci in the 3D nuclear space, has opened new avenues for studying chromosomal interactions.
3C technology
Job Dekker developed the 3C technology in 2002 to study the yeast system. This technology is chiefly used for detecting interactions between specific and adjacent DNA loci. Spatially adjacent chromatin fragments are crosslinked, and restriction endonucleases digest the crosslinks. A T4 DNA ligase preferentially ligates proximal DNA fragments. The relative abundance of ligated fragments is determined by quantitative polymerase chain reaction (qPCR).
4C technology
Circular chromatin conformation capture (4C) technology was developed to screen fragments interacting with the target at the genome level. In 4C, the two cross-linked DNA molecules are circularized, and an inverse PCR is performed using primers specific for the target DNA. With 4C, the interaction of a specific fragment with all possible sites could be determined using one set of primers.
5C technology
The chromatin conformation capture carbon copy (5C) technology was developed to capture multi-locus to multi-locus interactions. In 5C, after the 3C library is generated, ligation-mediated amplification is performed in a multiplex PCR to create a 5C library. The interactions between multiple sites could be assessed simultaneously using multiplexed primers and next-generation sequencing.
Hi-C technology
Dekker’s group developed the high-throughput chromatic conformation (Hi-C) technology for high-throughput analysis of chromatic interaction. Hi-C, based on 3C, involves the biotinylation of fragments after enzymatic cleavage. Magnetic beads coupled with streptavidin are added to enrich the fragments, and high-throughput sequencing is performed to obtain information on genome-wide interactions.
3D genome and viral infections
Severe acute respiratory syndrome coronavirus 2 (SARS-CoV-2) has caused severe public health and economic crisis. Lately, Hi-C technology has been applied to study the global 3D changes after infection in A549 cells expressing angiotensin-converting enzyme 2 (ACE2).
Studies revealed that host chromatin was remodeled to a large extent. Moreover, the active region of chromatin began attenuating. The contacts within topologically associating domains (TADs) were reduced. Consequently, inflammatory gene expression was upregulated, and interferons were downregulated, contributing to the disease pathology.
Researchers used Hi-C technology to identify more than 15,000 contacts/interactions between human and Epstein-Barr virus (EBV) genomes. Recently, the interactions between minute virus of mice (MVM), a parvovirus, and host genome were investigated using viral 3C (V3C) technology. They found that the MVM genome localizes to the DNA damage response region of the host genome to facilitate viral replication/infection.
The 3C technology was applied to understand the pathogenesis of human papillomavirus (HPV) in HeLa cells, wherein the viral genome is integrated into host chromosome 8. It was noted that HPV could influence MYC gene expression through long-distance interaction, thereby promoting cancer progression.
Another study using Hi-C technology revealed that the host genome could be disrupted by HPV by dividing one TAD into two. Besides, a team of researchers reported that HPV integration into the host genome alters the host genomic structure by introducing a new binding site of CCCTC-binding factor (CTCF), resulting in multiple changes in chromatin accessibility and gene transcription advancing tumor progression.
Concluding remark
While the application of 3D genome technology to explore therapeutics has been increasing, several limitations must be addressed. Notably, 3D genomics is chiefly centered around DNA and proteins, and only a few studies have involved RNA. Therefore, novel approaches are required to characterize the interactions among DNA, RNA, and proteins. Overall, 3D genome technologies could provide valuable insights into the changes in chromatin structure before and after viral infection and help to identify the critical interactions between regulatory elements and target genes.
- Liang W, Wang S, Wang H, et al. When 3D genome technology meets viral infection, including SARS‐CoV‐2. Journal of Medical Virology. doi: 10.1002/jmv.28040 https://onlinelibrary.wiley.com/doi/10.1002/jmv.28040
Posted in: Medical Science News | Medical Research News | Disease/Infection News
Tags: ACE2, Angiotensin, Angiotensin-Converting Enzyme 2, Cancer, Cell, Cell Division, Chromatin, Chromosome, Chromosome 8, Coronavirus, Coronavirus Disease COVID-19, DNA, DNA Damage, DNA Replication, Enzyme, Epstein-Barr Virus, Frequency, Gene, Gene Expression, Genes, Genetic, Genome, Genomic, Genomics, HeLa Cells, High-Throughput Sequencing, Interferons, Ligase, Ligation, Locus, Magnetic Beads, Parvovirus, Pathology, Polymerase, Polymerase Chain Reaction, Public Health, Research, Respiratory, Restriction Endonucleases, RNA, SARS, SARS-CoV-2, Severe Acute Respiratory, Severe Acute Respiratory Syndrome, Syndrome, Therapeutics, Transcription, Tumor, Virology, Virus, Yeast
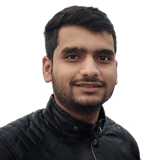
Written by
Tarun Sai Lomte
Tarun is a writer based in Hyderabad, India. He has a Master’s degree in Biotechnology from the University of Hyderabad and is enthusiastic about scientific research. He enjoys reading research papers and literature reviews and is passionate about writing.
Source: Read Full Article